Condensed matter physics
Condensed matter physics identifies the physical properties of condensed phases of matter. The phenomenon of electrons, atoms, spins, or other various components inside a solid material is described through quantum mechanics. These described phenomena are predicted through theory and calculation and proved through experiments. Therefore, theory, calculation, and experiment are very important factors in Condensed matter physics.
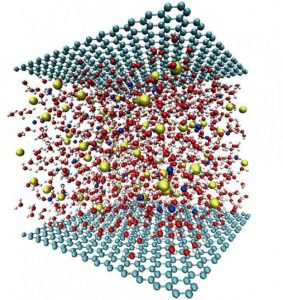
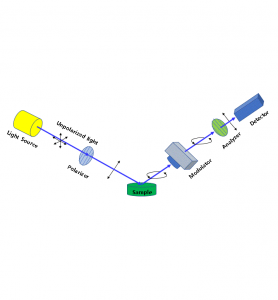
Optical Spectroscopy of Quantum Materials
Our research interest lies mainly on novel quantum materials with exotic optical properties. For this we measure optical transitions of various quantum matters such as Dirac-Weyl semimetals, 2d materials (graphene and TMDC), and strongly correlated transition metal oxides, utilizing wide-range infrared /optical spectrometers. We also characterize 2D material-based optical devices such as photoconductor and field effect transistors.
Prof. Seo’s team focuses on the fabrication of organic and inorganic electronic devices such as solar cells, field-effect transistors (FET), and organic light emitting devices. Light emitting transistors (LETs) are an emerging class of optoelectronic devices that combine the electrical switching functionality of a FET with the capability of light generation typical of light-emitting diodes (LEDs). Due to this unique combination of properties, LETs have potential applications in the fabrication of simplified pixels for flat panel displays, optical communication devices. Organic and perovskite solar cells are types of flexible solar cells that produce electricity from sunlight using solution processible materials. In order to improve the efficiency of devices, we are focusing on the materials design, self-assembly of donor and acceptor phases, device fabrication engineering and interactions at the photoactive semiconductor interfaces. Our research bridges areas of chemistry, material science, electronic engineering and physics.
Organic and Inorganic Semiconducting Materials and Electronic Devices
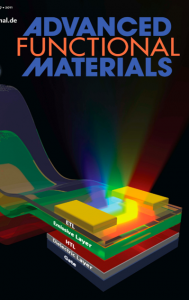
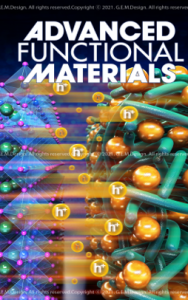
Photoelectron spectroscopy
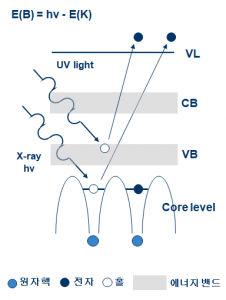
Photoelectron spectroscopy provides a wide range of information on nanoscale materials. When a sample is exposed to light of energy hv, electrons gain kinetic energy while following the law of conservation of energy. The value of the kinetic energy of the electrons emitted from the sample is calculated using Einstein’s photoelectric effect, and the binding energy is also measured. The measured binding energy represents the intrinsic energy of the element and varies depending on the chemical state, thus providing information on both elemental analysis and chemical state. X-ray photoelectron spectroscopy, which uses X-rays as a light source, and ultraviolet photoelectron spectroscopy, which uses ultraviolet light, are common types of photoelectron spectroscopy.
Nano Structure Analysis and Application
Nano Structure Analysis and Application Laboratory (NSAL) focuses on fundamental properties and applications of low-dimensional materials such as quantum dots and two-dimensional materials. We fabricate the low-dimensional materials using chemical methods and sputtering. We are interested in their optical, electrical, opto-electronic properties and electronic structure. Photoluminescence, Raman spectroscopy, I-V characteristics, and photoelectron spectroscopy are our main analysis techniques. We investigate a new application of the materials such as solar cells, photodetectors, LEDs, and transistors.
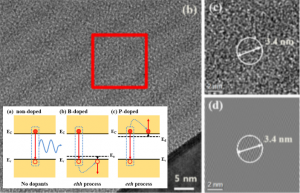
Electronic Structure of Thin Film
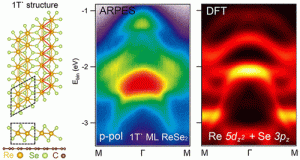
We investigate the unexplored characteristics of various material through thin film research. To study material properties, we synthesize thin film, especially high quality epitaxial thin film, using ultra-high vacuum deposition chamber. The materials we mainly study are metal chalcogenides, and we use x-ray spectroscopy(XPS, XRD, ARPES) to study electronic structure of thin film and surface-interface interaction. Specifically, we employ x-ray synchrotron accelerator experiments at PLS(Pohang Light Source, Pohang) and ALS(Advanced Light Source, Berkeley) to study materials in a more suitable energy range. In addition, we investigate electrical transport, device properties and electrochemical reactivities. We gain a deeper understanding the characteristics of materials and contribution to the development of advanced technologies through these experiments about applications.
Terahertz Biomedical Science and Applications
Terahertz Biomedical Laboratory covers recent advancements in terahertz imaging for medical applications, especially cancer diagnosis. The ultimate goal is to review the current state of THz imaging technology for diagnosing cancer and to explore the potential medical applications of THz radiation. We also consider the development of future medical applications using terahertz technology.
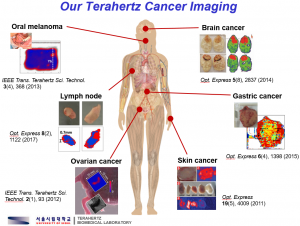
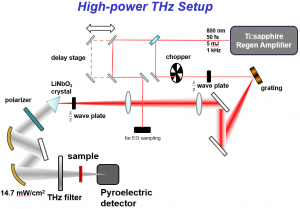
A number of applications including scientific spectroscopy, security screening, and medical imaging have benefited from the development and utilization of emerging terahertz (THz) generation and detection techniques. This major is the study of terahertz technology and exploration of basic biological research conducted using terahertz waves.
Computational Materials Research Group
Computational Materials Research Group at our university is a diverse team of researchers focused on studying various physical observables across different systems. Our group places a significant emphasis on 2D layered materials, including graphene, hBN, transition metal dichalcogenides, and their stacked combinations. By leveraging a range of computational tools such as Density Functional Theory, tight-binding methods, continuum models, molecular dynamics, and a multiscale combination of these approaches, we can approach each problem with the necessary precision and scale. Our ultimate goal is to use the most accurate models possible to allow for quantitative comparisons with and predictions for experiments, which has led to fruitful collaborations with top experimental groups both in Korea and around the world. Our work on layered materials has helped us provide an understanding of a range of
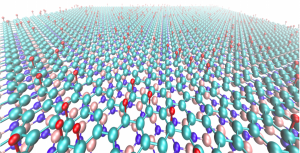
phenomena, including flat band physics in systems such as magic angle twisted bilayer graphene and moire physics in GBN due to the twist and lattice degree of freedom. Join us as we continue to push the boundaries of our understanding of condensed matter physics.